The next generation
19 Aug 2014
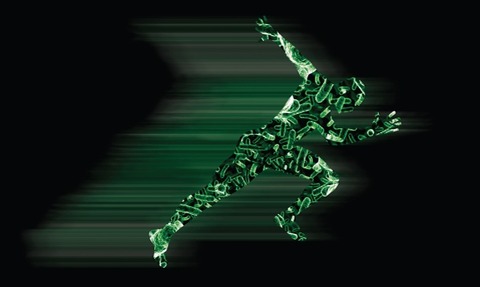
Nabsys vice president, marketing & business development, Darren Lee, tells LaboratoryTalk how electronic detection can complement next-generation sequencing (NGS).
With the introduction of Sanger and Maxam and Gilbert DNA sequencing technologies in the late 1970s, the ability to sequence an individual’s DNA took a great leap forward.
This led researchers to improve technologies by focusing on reducing error rates, increasing read lengths and making protocols robust enough for automation. Decades of improvement have made sequencing much less expensive and powerful enough to sequence the human genome.
“The three biggest features that researchers account for are cost per gigabase (Gb), read-length and error rate
Nabsys VP Darren Lee
Similar to the first generation of sequencing, NGS has improved dramatically since its introduction.
Throughout the process of NGS optimisation, the various commercial technologies offer different features and trade-offs that users take into account depending on their targeted application.
The three biggest features that researchers account for are cost per gigabase (Gb), read-length and error rate. Frequently, researchers could not obtain the best of all features for their application and would choose the performance combination that best suited their needs.
Usually, users would default to the least expensive sequencing method available at the time. The decision became more difficult, however, as the price per data point for sequencing continued to rapidly fall.
Fortunately, the NGS price free-fall has slowed so that investigators have been able to look more closely at factors beyond cost.
New instrumentation and the likelihood of new nanotechnology platforms have moved in to take advantage of the needs of particular segments of the sequencing market.
Issues with NGS data are of particular importance in areas such as clinical research and as sequencing moves further into molecular diagnostics.
Next-generation techniques rely on short-read data that is difficult to assemble, resulting in data gaps, misalignment and incorrect sequences. Next-generation techniques attempt to reconstruct genome data from millions of short-read fragments.
The contiguous sequences of DNA representing a consensus sequence assembled from overlapping short reads are called ’contigs’.
Contigs assembled from short reads, result in incomplete representations. In the absence of long-range structural information, next-generation technologies are limited to the analysis of short sequence variants.
Where NGS platforms provide the short-read sequence information enabling the understanding of small variants, additional technologies are required to provide the long-range structural information that is important in the study of diseases such as cancer and cytogenetics.
Furthermore, and most important in clinical sequencing, is time-to-results.
Current NGS data can take up to a week to generate and then additional time to analyse the vast collection of data generated. The time-to-results needs to be reduced dramatically for NGS to succeed in a clinical setting.
Several nano-detection platforms such as those developed by Nabsys have been discussed recently for their promise in addressing the two biggest short-comings with NGS data: short read lengths and time to results.
These nanotechnologies use either biological or solid-state nano-pores and electronically detect labelled or unlabelled DNA as it passes through the detector.
A major advance in solid-state nano-pore development includes Nabsys, who is pioneering the development of semiconductor-based tools for genomic analysis.
The main advantage of these single-molecule detection technologies is that they can utilise long read length of input DNA, often up to hundreds of thousands of kilobases in length.
In the study of cancer, issues can often arise due to heterogeneity and aneuploidy. As these technologies are ’single-molecule’ detection systems, they can detect a single signal from a mixed sample of cancer cells, decreasing the issue of heterogeneity.
“Current NGS data can take up to a week to generate and then additional time to analyse the vast collection of data generated
Nabsys VP Darren Lee
In addition, aneuploidy genomes result from the many duplications, deletions and chromosomal rearrangements that occur causing variation in the number of copies of specific regions of chromosomes, or entire chromosomes.
Often these rearrangements are small and difficult to identify through current technologies such as array Comparative Genomic Hybridisation (aCGH) or NGS yet not large enough to detect using classic cytogenetic techniques such as karyotyping.
But because of the large input sample enabled by these nanotechnology platforms, rearrangements, even balance translocations and inversions can be identified.
Improved understanding of the mechanisms behind diseases in areas such as cancer and cytogentics will greatly improve as our ability to study and understand the creation and function of large-scale structural variations in these disease areas.
Similar to how the advent of DNA microarrays dramatically improved our understanding of small-scale structural variants through Genome-Wide Association Studies (GWAS), technologies that enable direct analysis of very large sample read lengths and can generate longer-range information in concert with current NGS data, will enable GWAS studies of large-scale structural variants in the human genome.
As a complimentary tool to NGS, the Nabsys technology, by utilising long-read lengths and single molecule detection, will enhance the researchers’ ability to identify and study large-scale structural variants in genomics research and molecular diagnostics.