Shining a light on disease
24 Nov 2014
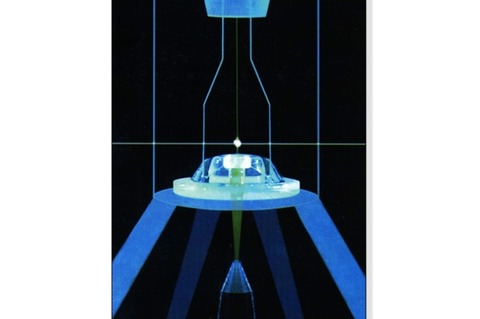
A more comprehensive awareness of flow cytometry technology could dramatically increase its utilisation by researchers at both smaller and more specialised laboratories.
Flow cytometry has established a strong foothold in large clinical and research laboratories, where it is used to analyse cells as they flow in a fluid stream through a beam of light.
“I rank it as a vital tool for research scientists,” says Divyaa Ravishankar, senior life sciences industry analyst at Frost & Sullivan.
The coupling of optical and electronic systems in flow cytometry helps determine the characteristics of cells by recording how they scatter light and emit fluorescence, offering information on their size, shape and other features.
“The market is really driven by pharmaceutical and biotech industries, with 80% of its application in research, and the remaining 20% for diagnostic purposes,” says Ravishankar.
It has an established place in both basic research and clinical trials because it allows laboratories to sort particles based on their properties to identify particular populations of interest, says Ravishankar.
“For example, if there were any agents for drug candidates that had lots of biomarkers, drug developers may turn to flow cytometry to study these.”
The market is driven by pharma and biotech industries, with 80% of its application in research, and the remaining 20% for diagnostic purposes
Frost & Sulivan life sciences analyst Divyaa Ravishankar
The capability to measure different parameters on a very large number of cells within very short periods of time is the key benefit of flow cytometry, she says, which can be divided into two broad categories: cell analysis and cell sorting.
Applications in life sciences include examination of gene and protein profiles, cell-based assays, pharmacogenomics, metabolic studies, stem cell research, and screening markers for gene expression, she says.
It also has a number of medical applications such as the diagnosis of haematological malignancies, lymphocyte enumeration, detecting residual disease or measuring the efficacy of chemotherapy or organ transplantation.
But in spite of its broad application, flow cytometry equipment is not a common fixture in smaller labs, says Ravishankar, owing to the costs and complexities of buying and maintaining systems.
“The price of a high- to mid-end instrument required for most research and clinical applications ranges from $50,000 (£32,000) to $500,000 (£320,000),” says Ravishankar.
Then there are the additional costs of running an experiment, such as consumables and maintenance expenses.
“The [unit] price varies according to the number of parameters that are being measured, and this makes sophisticated equipment largely the domain of larger laboratories who service smaller labs when required,” she says.
In addition, a lack of awareness of its many possible applications, has also inhibited more mainstream adoption, she adds.
Manufacturers of flow cytometry equipment include BD Bioscience, Beckman Coulter and EMD Millipore, and cell-based flow cytometry accounted for the largest share of the global market in 2013, says a report published by US researcher MarketsandMarkets.
“However, bead-based flow cytometry is expected to grow at a higher rate as compared to the cell-based ones because of decreased system cost, better statistical performance, and enhanced hybridisation kinetics,” the report says.
In the UK, flow cytometry is concentrated in very specialist laboratories, says Mandy Campbell, product manager for Horiba Medical, which is involved with flow cytometry and its application in the field of haematology.
“There are many instruments that use flow cytometry and the main reason we use it in haematology is its ability to look at each cell individually. It allows you to get characteristics of each cell as a way of differentiating normal and abnormal cells,” she says.
This could be used in the diagnosis of a new case of leukemia or detecting whether or not patients are in remission after treatment, she says.
It can also be applied to the measurement of drug uptake, because DNA is the site of action of many chemotherapeutic drugs.
“Flow cytometry is great for getting accurate results within these applications,” says Campbell.
While flow cytometry technology is now well established in specialised settings such as these, some new technological developments could boost its application substantially, says Ravishankar.
These include ’combination systems’, which blend the visual power of microscopy with flow cytometry in a single process to boost quantitative imaging, and ’multiplexing’ used in the xMap technology developed by US-based biotechnology firm Luminex, and can look at different targets at same time.
“Another is what I call microcapillary cytometry, which offers a compact throughput and automated cell count,” says Ravishankar.
“This can accommodate smaller volumes of samples than other traditional flow cytometry techniques that need sheath fluid. Microcapillary cytometry devices don’t need sheath fluid, which like reagents, are very expensive.”
With one of the biggest recurring costs in flow cytometry being reagent sales, Ravishankar says that any instrument that can conserve costs of reagents would be beneficial.
“I see microcapillary instruments being able to do that in the future, and they will also be easier to set up and maintain,” she says.
Massachusetts Institute of Technology (MIT) scientists have also been working on a type of microscopy that allows them to detail the interior of a living cell in three dimensions, without adding any fluorescent markers or other labels.
This year, the researchers said they had adapted that method to allow them to image cells as they flowed through a tiny microfluidic channel.
“Many stem cell applications require sorting of cells at different stages of differentiation. This can be done with fluorescent staining, but once you stain the cells they cannot be used.
“With our approach, you can utilise a vast amount of information about the 3-D distribution of the cells’ mass to sort them,” says Yongjin Sung, a former postdoc in the MIT’s Laser Biomedical Research Centre.
Instead of using fluorescent tags, the MIT method analyses the cells’ index of refraction - a measurement of how much the speed of light is reduced as it passes through a material.
Every material has a distinctive index of refraction, and this property is used, along with cells’ volume, to calculate their mass and density.